“Houston…we’ve had a problem here.”
A variation of that message, one of the most understated in space travel history, was sent by astronauts Jim Lovell and Jack Swigert to Mission Control at the NASA Space Centre in Houston on Monday, April 13, 1970. Those cryptic, now famous and unforgettable words, uttered when the Apollo 13 space mission ran into trouble far from Earth, have since entered the English lexicon as being synonymous with potential, impending disaster.
Apollo 13 had blasted off two days earlier, on April 11, 1970, aboard a Saturn V rocket, carrying a Command/Service Module and a Lunar Excursion Module for what was intended to be the third manned landing on the moon. If not a routine procedure by then, it was at least a familiar one. At 363 feet – the height of a 36-storey building – the Saturn V was the tallest and most powerful NASA rocket ever made and was intended to send people to the moon.
Its chief architect was Werner von Braun, known as the “Father of Rocket Science,” who began his career in Germany in the 1930s and early 40s developing the Nazis’ rocket program. This culminated in the V-2 rocket used by Nazi Germany to blitz England in 1944.
By the 1960s, von Braun had become a key figure in NASA’s development of the Saturn V rocket and the Apollo program announced under the presidency of John F. Kennedy. In a speech to Congress on May 25, 1961, Kennedy set as a national goal for America “landing a man on the moon and returning him safely to the Earth” by the end of the 1960s.
Perhaps less noticed by the public was an announcement just over one year later, on July 11, 1962, by von Braun that the Apollo program would use as its mode of propulsion the Lunar Orbit Rendezvous (LOR) system. The LOR system called for a Saturn V rocket to launch a spacecraft composed of a Command/Service Module (CSM) which would orbit the moon, and a Lunar Excursion Module (LEM) which would descend to the moon and later return to dock with the CSM. The LEM would be jettisoned into space before the astronaut’s return to Earth.
The LOR system had a huge safety advantage over a competing system which had initially been favoured by NASA. This competing system was known as Direct Ascent, whereby a spacecraft would land on the moon and then return to Earth.
Under the LOR system, only the LEM would descend to the moon and later return to dock with the CSM, allowing the astronauts who had descended to the moon to transfer back to the CSM. At that point the LEM would be jettisoned prior to the return journey to Earth. The advantage of LOR having both a LEM and a CSM – rather than the one spacecraft envisioned under the Direct Ascent model – was that the LEM could be used as a “life boat” in the event that there was a catastrophic failure aboard the CSM. This was a touch of fortunate prescience on the part of NASA scientists.
Of course, UTIAS personnel had no idea back on July 11, 1962, when von Braun made his announcement in favour of the LOR system, that such a massive failure would occur eight years later during Apollo 13. Nor did they have any inkling that NASA would call on UTIAS expertise which would lead to a “Made in Canada” solution that helped save the lives of the three American astronauts.
The Big Bang
The first indication of a problem occurred on the evening of Monday, April 13, 1970 when the Apollo spacecraft – composed of the CSM called Odyssey joined by a tunnel to the LEM called Aquarius – was 200,000 miles from Earth and closing in on the moon. Mission Control in Houston noted a low-pressure warning signal on a hydrogen tank in Odyssey.
But the astronauts aboard the craft – first-time flyer and CSM pilot Jack Swigert, 38; flight commander Jim Lovell, at 42 the most experienced astronaut with three previous space missions under his belt, and LEM pilot Fred Haise, 36 – didn’t need an instrument reading to know something had gone terribly wrong. They heard a heart-stopping bang as the tank, filled with liquid oxygen, exploded. Their lives hung in the balance.
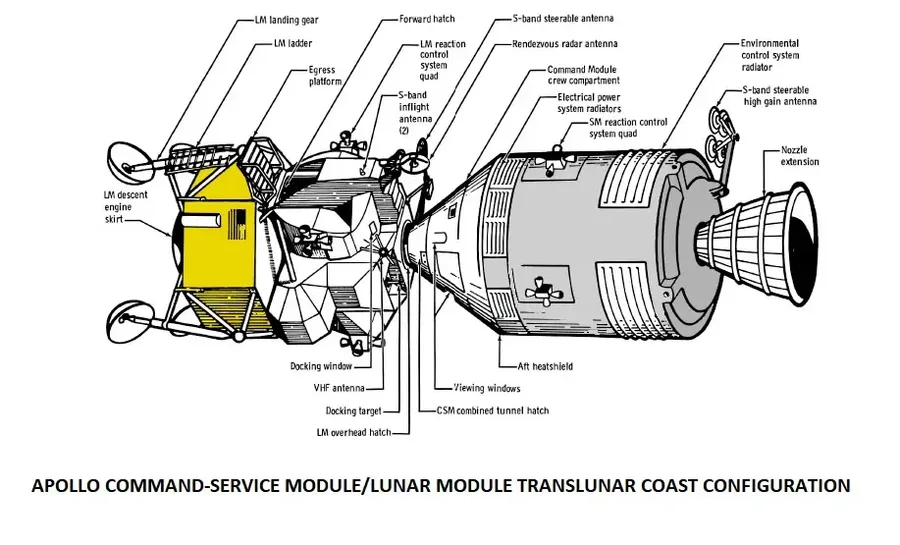
Image credit: NASA
Mission Control in Houston, where NASA flights are monitored and controlled, immediately began crash analysis and information-gathering, working in conjunction with the Kennedy Space Center at Cape Canaveral, Florida, where the launch site was located.
Canadian Press reported in 2010 that Dr. Bill Carpentier, a retired NASA flight physician, was in the Mission Control room when Lovell and Swigert informed ground controllers in Houston there was a problem. The Canadian-born doctor was monitoring the astronauts during their April 1970 flight. He said it became immediately clear at the time of the explosion that the problem was with oxygen levels inside the CSM.
“As soon as they knew they had a problem, you could see on the electrocardiograms their heart rates went up considerably and their respiration rates went up," the B.C. native said in an interview with CP’s Peter Rakobowchuk.

Image credit: NASA
Apollo 13 Flight Journal Day 3: Aquarius Becomes a Lifeboat
UTIAS…we have a problem
Five days after launch and three days after the on-board explosion, a call came from Grumman Aerospace to Prof. Barry French, a senior scientist and expert in gas dynamics at UTIAS in Downsview, Ontario. Barry had previously worked with a number of NASA scientists and contractors, including some at both Martin Marietta and Grumman Aerospace, who were joining with NASA in asking for help from UTIAS.
Professor Phil Sullivan wrote: “On Thursday, April 16th, 1970 we were absorbed in the minutiae of a departmental meeting when a secretary interrupted, informing us that an engineer…had called Professor Barry French to help with the rescue of Apollo 13. The meeting broke up and French assembled a team of advisers from the…(UTIAS) faculty.”
My colleagues and I at UTIAS had all followed this mission as best we could at the time, much as we kept an eye on most of NASA’s activities. This was long before the invention of the Internet.
The mission was going quite well as far as we knew, so we were shocked to hear BBC news reports on April 14, 1970 about a critical explosion the previous day that had crippled the spacecraft. One of the BBC reports said: “An explosion on board Apollo 13 has caused one of the most critical situations in American space history and put the lives of the three astronauts on board in severe jeopardy.”
We understood that around 8 p.m. on Monday, shortly after the Apollo 13 public television broadcast signed off, the astronauts heard a large bang accompanied by some vibrations. The actual wording of the message sent by Swigert to NASA Mission Control around 9 p.m. that night was (from the Apollo 13 Day 3 Journal):
055:55:19 Swigert: Okay, Houston...
055:55:19 Lovell: ...Houston...
055:55:20 Swigert: ...we've had a problem here. [Pause.]
Initially, the astronauts thought that the spacecraft had been hit by a meteor, but we later learned from the BBC report that an “explosion happened in the fuel cells of the spacecraft's Command/Service Module approximately 55 hours after liftoff. This resulted in the loss of Apollo 13's main power supply which means oxygen and water reserves are now critically low.” This occurred when Apollo 13 was about 200,000 miles from Earth and travelling at 2,100 m.p.h.!
I was in my office when Barry French appeared at my office door. He asked whether I could join him and other colleagues in the UTIAS auditorium – a large lecture hall with a blackboard, useful for on-the-fly calculations – as soon as possible to help analyze the Apollo crisis.
At that time, I had just been promoted to associate professor status with tenure (the much sought-after achievement of all professors!) and my research focused on structures, a relatively new field of aerospace which looked at materials and their characteristics, including “shell” and “plate” structures. Shell structures included cylindrical shapes, such as the body of the Saturn rocket, and plate structures looked at panels used to construct satellites and aircraft.
I was working on my new NASA proposal to study the effects of the space environment on advanced composite materials such as carbon/epoxy using a space simulation chamber on loan to me from Barry. (His generous donation to my project made this research possible.) These were relatively new materials not used by the Canadian aerospace industry at that time, but they were under investigation by NASA and the U.S. Air Force for applications in spacecraft and fighter aircraft. These materials seemed highly suitable due to their light weight, compared with aluminum, and their relatively high strength and stiffness.
The Dream Team
The UTIAS team assembled by Barry French possessed a unique combination of expertise in a range of specialized fields uncommon anywhere in North America. Barry, in addition to being the go-to guy for Grumman on this problem, was originally a chemical engineer and had done a master’s degree in thermodynamics. He had worked with Orenda Engines, a Canadian aircraft engine manufacturer, part of the Avro Canada conglomerate which between 1946 and 1962 produced the prototype of the CF-105 Arrow, one of the most advanced interceptor aircraft in the world. The Progressive Conservative government of John Diefenbaker cancelled the (Avro) CF-105 Arrow development in 1958, a controversial decision which still arouses debate to this day. Barry, who had completed his doctoral thesis at UTIAS in theoretical and experimental plasma diagnostics, also worked in the ramjet division of an English turbine company.
When I arrived at the auditorium, I was met by Barry and by Professor Ben Etkin, well-known as a global expert in aircraft guidance and control, aerodynamics and satellites, with particular expertise in model analysis. Ben had worked on the design and production of gliders, as well as the iconic Avro Arrow and de Havilland aircraft during and after the Second World War, including research on hypersonic flight and simulations of atmospheric re-entry.
In addition, three other UTIAS scientists were gathered in the auditorium. Professor Irving Glass was a gas dynamics and shock wave specialist. He was responsible for building Canada’s largest shock tube facility for investigating hypersonic flight, and a sonic boom facility for studying supersonic shock wave effects on buildings and structures. His knowledge of shock wave effects on structures was crucial to this analysis.
Professor Phil Sullivan was a fluid mechanics specialist and Canada’s premiere expert on the design and flight mechanics of air cushion vehicles (ACVs). He built, at UTIAS, the only enclosed circular track facility for testing models of ACVs.
Professor Peter Hughes was a satellite, orbital mechanics and robotics expert, one of the key pioneer developers of the Shuttle Remote Manipulator System (RMS). Usually referred to as the Canadarm, the RMS was one of the primary space tool fixtures on NASA’s later vehicles, the space shuttles.

The UTIAS Apollo 13 team in the LH in 1970: from left: Rod Tennyson, Irv Glass, Phil Sullivan, Ben Etkin, Barry French (absent: Peter Hughes)
I was fascinated and rather excited to hear what Barry had to say, and why we were there.
"Some of my colleagues and I,” Barry French later recalled, “were phoned by NASA and they wanted advice. The Lunar Excursion Module was their life boat. It had the life support stuff in it that allowed them (the astronauts) to breathe and have a little bit of battery power, but near the end of the flight back to Earth, they had to separate the re-entry component from this lunar module in a way that wasn't planned for."
The Lunar Excursion Module was never designed to support three astronauts for an extended period, that was the job of the Command/Service Module, but after the explosion, it was not serviceable. All the components of the spacecraft were still connected, including the now-damaged CSM. Keeping the astronauts alive while working out a way to detach the LEM — which was never designed to return to Earth — from the CSM was clearly going to be the primary issue to resolve.
The Magic Number
As we gathered in the auditorium, we were given to understand that NASA had a particular critical calculation it was having difficulty with and had contacted a number of the major aerospace and engineering organizations across North America to all work on the problem simultaneously. These groups ostensibly included such renowned organizations as MIT, Stanford University, CalTech (the California Institute of Technology), Georgia Tech and, of course, NASA Langley, NASA’s structures laboratory.
But time was running out; the explosion had occurred three days earlier and the countdown to catastrophe had begun. We — and we believed, all the other groups working on this problem — had to provide NASA an answer to their dilemma that day because the actions necessary to save the astronauts’ lives had to be done within the next 24 hours. They needed our calculations and our answer within hours.
To understand the problem we were tasked with solving, one needs to understand exactly what NASA needed: what had happened to the structure of the Apollo spacecraft and it’s amazing in-space reconfiguration that made the return journey so hazardous and how did NASA plan to rescue the astronauts?
The crucial calculation that NASA needed that day from the experts at UTIAS was a number — one single number — a calculation of the correct amount of air pressure which could safely separate the two components of the damaged spacecraft: the Lunar Excursion Module from the Command module, following separation of the damaged Service module, prior to re-entry. That separation would allow one component, the Command Module, to safely return to Earth in one piece with the astronauts and avoid burning up on re-entry into Earth’s atmosphere.
Calculating that one number involved understanding several issues, including the Saturn rocket configuration, a planned manoeuvre which changed the structure and its component parts during its approach to the moon; as well as the tunnel area which connected the components that had to be separated. We also had to “guesstimate” the damage done to various parts of the spacecraft by the explosion, and the strength of the hatch on the Command Module.
Understanding the Apollo Structure
NASA has a horizontal display of one of its massive, 363-foot-long Saturn rockets at its Cape Canaveral launch site in Florida. By comparison, the reusable Space Shuttle developed years later was about 121 feet long, one-third the size of the Saturn V.
On the Apollo 13 mission, the Command/Service Module (CSM) was made up of two parts. The Command Module (CM) at the top of the rocket was a cabin which could house a crew of three and was the only part of the rocket designed to return to Earth. So, it was the only section of the spacecraft protected by heat shields and which contained equipment designed for navigation, life support, re-entry and splashdown. Below the Command Module was the Service Module (SM) which provided primary life support equipment, electrical power and materials required during the mission, together with the problematic oxygen tanks. The oxygen tanks provided not only air for the astronauts to breathe but also powered fuel cells for electrical power for the Command Module’s many systems. The SM was designed to detach after the mission and burn up during re-entry.
Below the Service Module was the engine/propulsion compartment complete with rockets and rocket fuel. At the base of the rocket was the Lunar Excursion Module, designed to land on the moon, complete its lunar mission, return the astronauts to the Apollo spacecraft and then remain in orbit around the moon until its orbit decayed, which would send it crashing back onto the lunar surface.
At launch, the Saturn V structure consisted of three rocket fuel/propulsion stages, or sections, at the bottom of the spacecraft which lifted the spacecraft off the landing pad and up to space altitude. Two large fuel/propulsion stages fell away sequentially after completing their ignition, burns and lifts. The third propulsion stage was designed to get the spacecraft up to the right altitude and orient it into the correct transfer orbit to the moon. It was after the firing of this third stage rocket and after the reconfiguration of the entire Apollo spacecraft that the explosion of the oxygen tank occurred, 55 hours into the flight.
Very Tricky Musical Chairs
Once in Earth orbit, the astronauts had to oversee a generally unknown, critical and complex reconfiguration of the spacecraft. In orbit and moving towards the moon, the astronauts basically had to play musical chairs with the spacecraft’s components.
The Lunar Excursion Module had to be moved from the bottom of the spacecraft to the top — or front — with its propulsion system pointing towards the moon. The remaining rocket propulsion module, at the bottom of the spacecraft after the reconfiguration, was intended to be fired up for propulsion and navigation, and eventually detached before Apollo returned to Earth.
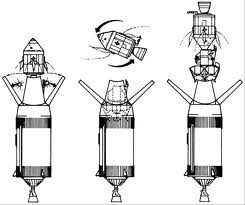
Image credit: NASA Apollo Project
Schematic of inflight manoeuvre of the CSM to attach to the LEM
This is a tricky manoeuvre. The Command/Service Module, connected together, was detached from the third rocket stage, rotated 1800 in orbit, and then reattached to the exposed connector docking device on the LEM, still contained within the protective shell of the third rocket stage. As shown in Figure 2, the outside panels of the stage 3 rocket component opened up, the CSM attached itself to the LEM and then pulled the LEM free of the last rocket stage, which fell away from the spacecraft and was subsequently propelled to impact the moon.
A view of the LEM still inside the expended third rocket stage is shown in Figure 3, photographed by the astronauts from the CSM as it approached its docking manoeuvre. The new Apollo configuration, now free of the shell of the last rocket stage, then again rotated in orbit so that the LEM was at the nose of the spacecraft and the rocket was at the base of the Service Module for mid-course corrections, as shown in Figure 4. A schematic drawing of the components of the Apollo configuration is shown in Figure 5.
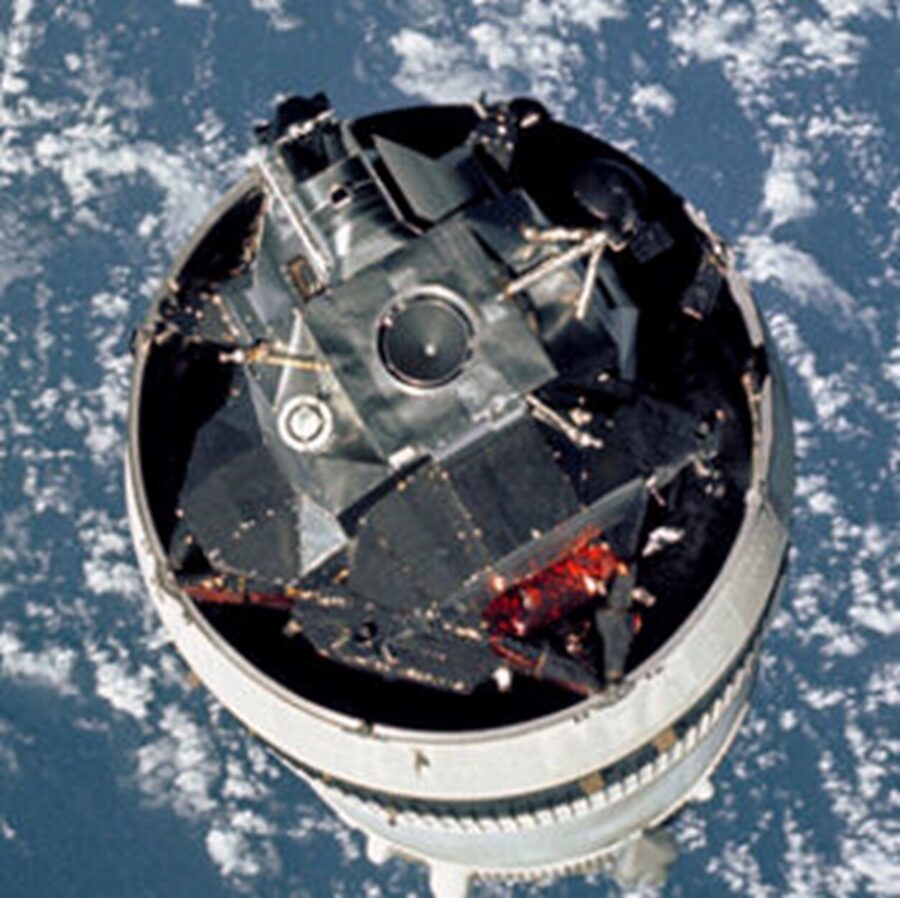
Photo credit: NASA
View of LEM in Saturn rocket stage as seen from the CSM
The Problem
It was after the orbital reconfiguration manoeuvre that the docking tunnel between the CSM and the LEM was connected, allowing the astronauts to move back and forth between the two modules.
The explosion of the oxygen tank negated the use of the fuel cells that generated the main spacecraft’s electrical power, heat, oxygen and water supply. NASA decided that the astronauts had no choice but to retreat to Aquarius, the Lunar Excursion Module, which would become their “lifeboat” for the duration of the mission and help get them home by the most efficient route, still allowing them to land in the Pacific Ocean. The docking tunnel, its hatch kept open for the remainder of the mission, would become the most problematic component of their return to Earth.
The LEM was designed as an operating base on the lunar surface and had its own separate power, propulsion and life-support systems. But it wasn’t designed as living quarters for all three astronauts and it wasn’t designed for re-entry into Earth’s atmosphere. The LEM was designed for two people with consumable supplies for about 60 hours.
NASA estimated that to survive up to re-entry, the three astronauts — Jim Lovell, Jack Swigert and Fred Haise — needed about 90 hours of supplies. They clearly had to conserve their oxygen and water, as well as improvise on the extraction of the carbon dioxide in the air using both the air filters in the LEM and a system rigged from the CSM to the LEM. Two astronauts would occupy the LEM while a third would stay in the CSM to monitor systems and maintain contact with ground control. Since no heat was available, the temperature dropped to 380 F, with lots of condensation from the astronauts’ breath accumulating inside the LEM and on the wiring.

Image credit: NASA
During a normal mission, the Command Module would be detached from the Service Module, as well as from the rest of the spacecraft, including the LEM, either prior to or during the return to Earth. But since the only propulsion available after the explosion was on the LEM, everything had to remain connected until the last possible moment.
“Normally, the LEM would have been jettisoned just after completing its mission by severing the tube (the tunnel) connecting it to the (CM) re-entry module,” Professor Phil Sullivan wrote on the University of Toronto website in April 2010, commemorating the 40th anniversary of the Apollo 13 rescue mission. “This tube, which also served as the LEM access tunnel, was to be cut by a ring of explosives located just four inches from the re-entry module's hatch. To ensure that shock waves from the explosion did not damage the hatch, before detonation, (in a normal Apollo mission) the 5 psi (pound-force per square inch) oxygen atmosphere in the tunnel would have been evacuated. The service module's rockets would then have been used to back away from the LEM. But because these rockets were inoperative, NASA's engineers proposed using the oxygen pressure (in the tunnel) as a spring to jettison the LEM just before re-entry.”
NASA knew, Sullivan recalled, that a previous incident indicated that retaining the full 5 psi in the tunnel on detachment could cause shock damage to the hatch. It was on this point, Sullivan said, that an engineer at the LEM manufacturer called UTIAS for advice.
Critical Calculation
Successful and safe detachment of the command module from the LEM prior to re-entry was the critical component in saving the astronauts — it could not re-enter Earth’s atmosphere dragging the LEM behind it. That would have knocked the Command Module off its re-entry path, and it could have been hit by any of the various components dragging behind it.
The connecting tunnel between the Command Module and the LEM was, in reality, simply a small corridor only 16 inches long and 32 inches in diameter. A ring of explosives located 10 centimetres – about four inches - from the hatch of the Command Module would normally have detached the rest of the spacecraft.
The team at UTIAS was asked to figure out exactly how much air pressure — in actuality, pressurized oxygen — was needed to separate the two modules, to detach the LEM from the Command Module without damaging either the Command Module or its internal tunnel entry hatch, which would be exposed to the atmosphere on re-entry.
"That short tunnel had air in it that was pressurized and when the ring of explosives was set off, the two parts of the returning spacecraft would be pushed apart by the pressure in that tunnel," UTIAS team member Ben Etkin told journalist Peter Rakobowchuk in the April 2010 story carried by Canadian Press. "If the pressure was too low, the two parts of the spacecraft would not be pushed apart with sufficient speed. Above a certain pressure, the shock wave caused by the explosive could damage the entry hatch to the re-entry module."
If the hatch had been damaged, said Etkin, it could have meant death for the astronauts during their return. "During the approach (to Earth), if the hatch was damaged and they lost air pressure inside the compartment due to leakage through that hatch, it would have been game over.”
The University of Toronto team was asked to figure out precisely how much pressure in that short corridor needed to be increased in order to separate the two modules, in conjunction with the explosive ring, without damaging the internal hatch.
Phil Sullivan described that day’s progress at UTIAS — Thursday, April 16, 1970 — in the 40th anniversary story which appeared on the University of Toronto’s website:
“With a telephone line held open to allow us immediate access to data on spacecraft geometry, masses and other quantities, we worked in two groups. One used Newton's laws of mechanics to estimate LEM separation speeds attainable with various tunnel pressures. The second group estimated the strength of the pressure pulse generated by the explosive charge. They adapted formulas verified in the first instance by comparisons with photographs of the first atomic explosion at Alamogordo, New Mexico.”
Being a “structures guy,” I needed to know what the proposed pressure pulse was in order to estimate the deformations that would, or could, occur on the hatch structure. So while these calculations were under way on separation speeds and tunnel pressure, I returned to my office to review plate models subject to lateral pressure pulses in order to estimate what deformations might occur and if they could possibly damage the hatch seal.
I was familiar with both the materials used and the strong honeycomb structure and plate material of the hatch, which was an aluminum sandwich structure, very stiff and not likely to undergo significant deformations under the pressure levels being discussed by the group.
I knew the air pressure in the connecting tunnel could be increased substantially without damaging the internal hatch. The concern was that the increased air pressure in the tunnel combined with the necessary detonation of the explosives ring around the far side of the tunnel might deform the internal hatch, making it difficult or impossible for the astronauts to close and seal it. I knew that wasn’t going to be an issue; the hatch would withstand the explosion and would retain its shape and integrity.
Professor Peter Hughes was also quite familiar with the Apollo program, and an expert on orbital dynamics and space trajectories.
“I had been leading the space vehicle dynamics and control research group at UTIAS for about five years when the Apollo 13 events transpired,” Hughes recalled 40 years later in the University of Toronto website story. “The Earth-plus-moon trajectories are much more interesting than simple Earth orbits, so I had also been using the Apollo baseline and backup trajectories as detailed examples in my UTIAS graduate lecture courses on orbital dynamics. When we all gathered around the big blackboard in the lecture room, I was able to explain to my colleagues the so-called ‘free return’ trajectory the astronauts were on. I had also been an invited commentator on both CBC and CTV during the Apollo launches, and in preparation for those, I had read dozens of NASA reports giving great detail on the Apollo designs, so I was able to contribute numerical design values (including geometrical and mass properties) for the Command and Service Modules.”
The UTIAS team, said Prof. French, “had to work off inadequate information as they sought to figure out what was needed to separate the two parts of the spacecraft using pressurized oxygen. Too little pressure, and the separating craft might have collided as they hit the Earth's atmosphere. Too much, and the lunar-module hatch would have been fatally damaged. We had to guesstimate things; it really was almost what you might say back-of-the-envelope.”
Coming Home
To get back home, Apollo actually had to navigate towards the moon, use its gravitational pull to sling-shot around it, and then use the propulsion system (descent engines) on the LEM to propel itself towards Earth.
NASA quickly realized that the normal navigation method using star shots — setting course by the stars in our galaxy — would not work because of the damage to the navigation system combined with interference caused by all the debris from the oxygen tank explosion that surrounded the spacecraft. Thus, they had to rely on navigation calculations based on what the astronauts could actually see: the Earth and sun, not the kind of navigation astronauts were accustomed to using. The stars in star-shot navigation don’t move; the Earth and the sun do.
About four hours prior to re-entry, the crew determined that it was time to separate the Service Module from the Command Module by triggering explosive devices. As it drifted away, the astronauts took photos of the damaged SM (as shown in Figure 6). The damage was extensive: an entire panel had been blown off the Service Module and a considerable amount of the equipment inside was gone or damaged. This can be seen by the missing outer panel of the module.
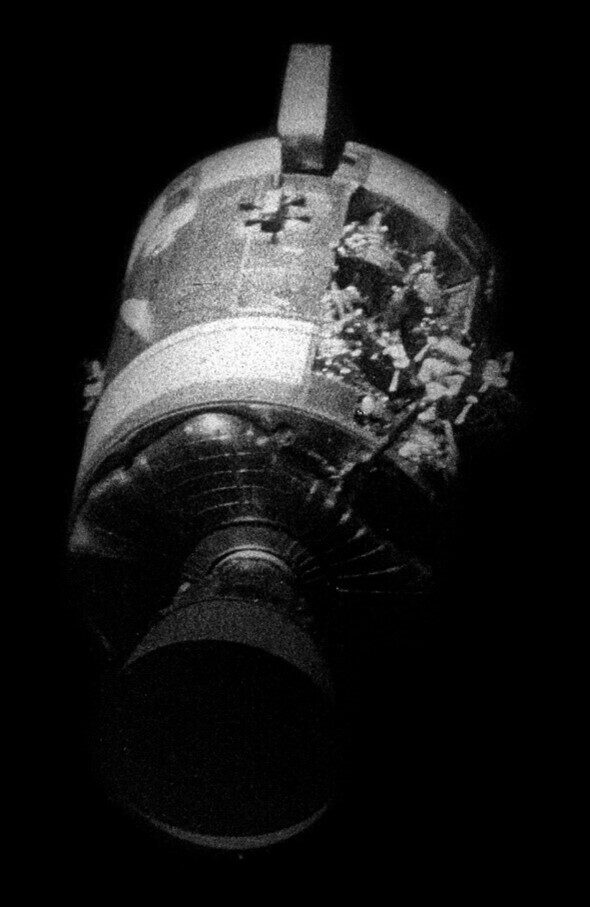
Photo credit: NASA
Photo of the Service Module with missing outer panel as it drifts away from the Command Module, which was still attached to the LEM
Due to the improvised navigation system, when the spacecraft entered the gravitational pull of the Earth, NASA computer models showed they would miss the Earth by about 99 miles and fly off into space! Another mid-course correction was needed and the only source for that was the LEM’s much smaller descent engine, never before tried for such a manoeuvre. They tried it and it worked.
About three hours later, it was time to jettison the LEM, power up the Command Module with its remaining fuel and get ready for re-entry. Figure 7 shows a photo of astronaut Fred Haise floating through the hatch connecting the tunnel between the LEM and the CM. To separate the LEM from the CM the astronauts would retire to the Command Module from the LEM through the connecting tunnel and close the adjoining hatch. They would use the explosive ring to detach the LEM and assume the internal pressure in the tunnel calculated by the UTIAS team would be sufficient to push the LEM far enough away, but not disturb the orbit and trajectory of the CM.

Photo credit: NASA
Fred Haise floating through the tunnel hatch connecting the CM and the LEM
The Answer
With time running out, the UTIAS team provided our answer by the end of that day. About two pounds per square inch of air pressure left in the connecting tunnel would be sufficient to push the LEM and remaining parts of the spacecraft far enough away from the Command Module for re-entry. And the internal hatch, which would be exposed to space after the detachment, was strong enough to remain intact, in the correct shape and sealed properly.
We delivered our calculations and conclusions to NASA by about 4 p.m. Thursday, April 16, 1970, as the astronauts began to prepare for re-entry the next day. At UTIAS we wrapped up our day and went home. As it turned out, that was about 20 hours prior to re-entry — another indication of just how fast we had worked out the calculations to determine the pressure level!

Schematic sequence representing separation of Service Module and Lunar Excursion Module from the Command Module prior to re-entry
Canadian Press reported in April 2010 that Bill Carpentier, who now lives in Belton, Texas, said “the most tense moments came during the re-entry into the Earth's atmosphere when there's usually a communications blackout. The blackout period was longer than usual and that made everybody very anxious. When finally they had communications with the spacecraft, they were on the right glide path to come in and you knew they were going to make it."
“It was the intuition and educated guess work that went into it that made it work,” said Barry French. “Next morning we woke up and heard that it had landed successfully and I remember feeling just amazed, relieved and happy. It was a bit awe-inspiring, actually.”
The hatch held and Apollo splashed down in the Pacific Ocean from where the three astronauts were plucked to safety on Friday April 17, 1970.
Post-flight Analysis
At UTIAS, the most astonishing fact about the rescue mission was revealed to us only weeks after the successful recovery.
□ We thought we were only one of a number of organizations and institutions calculating the critical issue of the air pressure needed to separate one component of the spacecraft from another, and the strength and integrity of the hatch. We were mistaken. In fact, we were the only ones NASA had called on to resolve that issue. Fortunately — and perhaps wisely — they didn’t tell us that. The pressure of that knowledge would have been enormous.
□ At the time, and even subsequently, there was no public mention made of the superstition around the reputed bad luck of the number 13. But the mission was Apollo 13 and the accident happened on April 13.
□ As for the cause of the explosion of that critical oxygen tank, a NASA review panel analyzed the explosion and a simple but detailed explanation was provided by Cape Canaveral Bureau senior producer Jim Banke on April 13, 2000. While a detailed explanation can be found on the space.com website, Banke described the origin of the problem which happened much earlier than the mission.
Five years earlier, in 1965, there was an overall design change in the electrical systems for the Command and Service Modules. Previously, the systems had all operated at 28 volts. In 1965, a design change mandated that all systems would be redesigned to operate at both 28 volts and at 65 volts.
“But one crucial participant never got word of the change,” Banke wrote. “Within the service module were two tanks of liquid oxygen. Oxygen from these tanks was used not only for the astronauts to breathe, but to help run three fuel cells that provided electrical power to run the command ship's many systems. Inside each oxygen tank was a thermostat which, along with a heater, was used to regulate the temperature inside the tank. It was the manufacturer of this thermostat that never learned of the need to accept 65 volts of electricity. All things being equal, that might not have been a problem. In fact, the oxygen tanks used on all previous Apollo missions had flown without trouble. But the Number 2 oxygen tank aboard Apollo 13 did have a slightly tarnished history.”
Two years earlier, the tank in question had been accidentally dropped about two inches by technicians handling it. In pre-flight testing before the launch of Apollo 13, the tank could not be emptied because the small filler tube had been damaged two years earlier. Technicians turned on heaters inside the tank to change the liquid oxygen to gas that could be vented. The thermostat inside, which was supposed operate at both 28 and 65, hadn’t been retrofitted; the 65 volt current was too high, the thermostat welded closed and the temperature inside the tank rose to 1,0000 F., which damaged wiring inside the tank. No one knew.
During the flight, astronaut Swigert was directed to turn on fans inside the two liquid oxygen tanks to mix the contents in order to ensure accurate readings. When the power was turned on in Tank 2 to heat and mix the oxygen, the thermostat contact points that were welded together because of the high 65 volt current load, didn’t control the temperature. This caused the tank to overheat to as high as 10000 F. Teflon insulation is known to ignite at such a temperature, and the fan-motor wiring exposed to the oxygen environment sparked. The oxygen inside the tank caught fire, the pressure increased and the tank exploded.
□ The Lunar Excursion Module was not equipped with sufficient carbon absorption material – it was designed for 40 hours of use, not 90 hours of living space for all three astronauts. The astronauts were in danger of poisoning themselves with carbon dioxide – their own breath.
After the explosion, there was also insufficient power for ambient heat and the LEM could only maintain a temperature of 38º. Because of the cold temperatures and the astronauts’ forced use of the LEM for living quarters, moisture from the astronauts’ breath was condensing on the electronics inside the LEM. They had to rig up a host system from the Command Module to the LEM to get rid of carbon dioxide inside the LEM while they lived in it.

Photo Credit: NASA
The crew of Apollo 13, Jim Lovell, Jack Swigert and Fred Haise, during a post-flight debriefing
Splashdown!
The next day, everyone at UTIAS and throughout NASA were jubilant, relieved that the astronauts had returned safely to Earth. None of us had any idea whether our work had actually played a part in saving the mission. It was only several days later that Barry French spoke with Grumman and learned that, in fact, Grumman had called only UTIAS to determine that critical calculation three days after the explosion. Barry shared that news with the team, and word spread throughout the institute.
We were very relieved that we had not been told in the heat of the crisis that the fate of the astronauts was solely in our hands. The pressure on all of us would have been enormous and could have led to some serious second-guessing at every step along the way. We had no computers at that time, no access or time for any computer modelling and no test data to work with; all our calculations were done on a blackboard and by slide rule, which is not accurate to very many decimal points.
As a “structures guy,” I was certain that the hatch and the structure would hold throughout the critical detachment of the modules. But as a junior associate professor at the time, I was enormously impressed that UTIAS was clearly so very well regarded by those in our field. And then the gold standard of authentication arrived at the institute just a few days later in early May 1970:
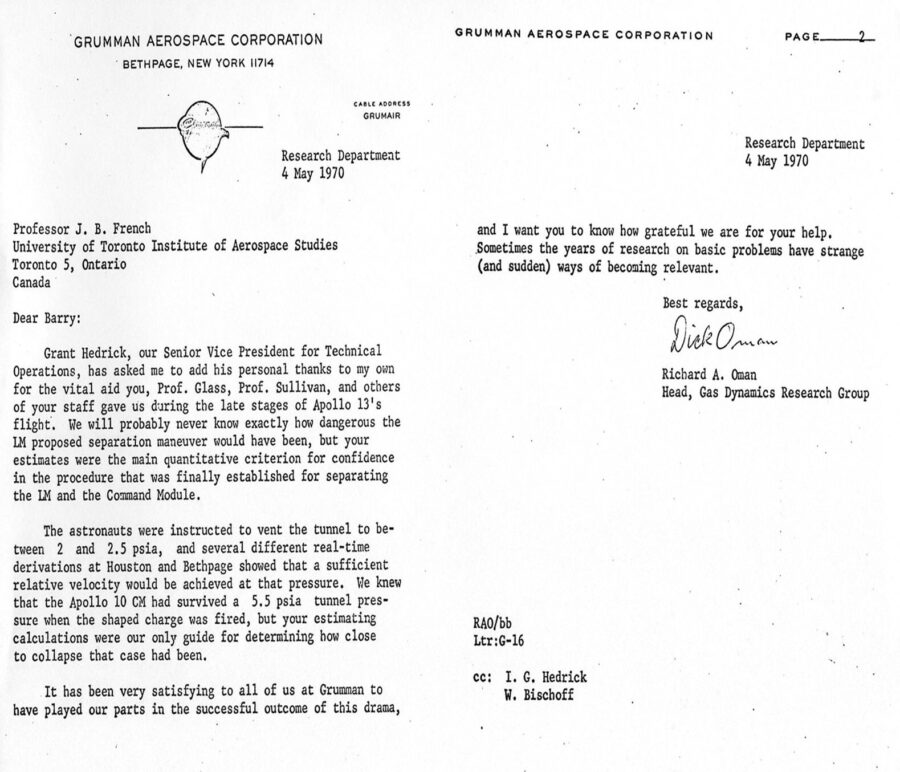
Canadian Recognition
Forty years after the accident that threatened the lives of the Apollo 13 space crew, our team of UTIAS engineers was recognized in February 2010 when the Canadian Air and Space Museum bestowed their Pioneer Award for the little-known role we played in averting a tragedy.
In accepting the Pioneer Award Professor Sullivan said “the team's largely unsung role in helping save the astronauts was unique and gratifying.” A letter from Apollo 13 astronaut Fred Haise Jr. was read out at the award ceremony in which the lunar module pilot praised the team's crucial help:
“Let me join others in congratulations to the University of Toronto Institute of Aerospace Studies as the recipient of this year’s Air & Space Pioneer Award. As the Lunar Module Pilot on Apollo 13, I would certainly vouch for their credentials. Their study and calculations provided a safe means to utilize pressure in the tunnel area between the Command & Service Module (CSM) and the Lunar Module (LM) to assure safe separation of the LM without damaging the hatch. This was another one of the new procedures that had to be implemented on Apollo 13 to assure our safe return. My sincere thanks and congratulations again on your well-deserved award!”

Accepting the Canadian Air & Space Museum Award in 2010: from left: Ben Etkin, Barry French, Phil Sullivan: Absent: Rod Tennyson, Irving Glass, Peter Hughes.
And, the UTIAS community gathered in the LH to watch The Honourable Ken Dryden, M.P. York Centre, read a Standing Order 31 in Canadian parliament to mark the occasion.
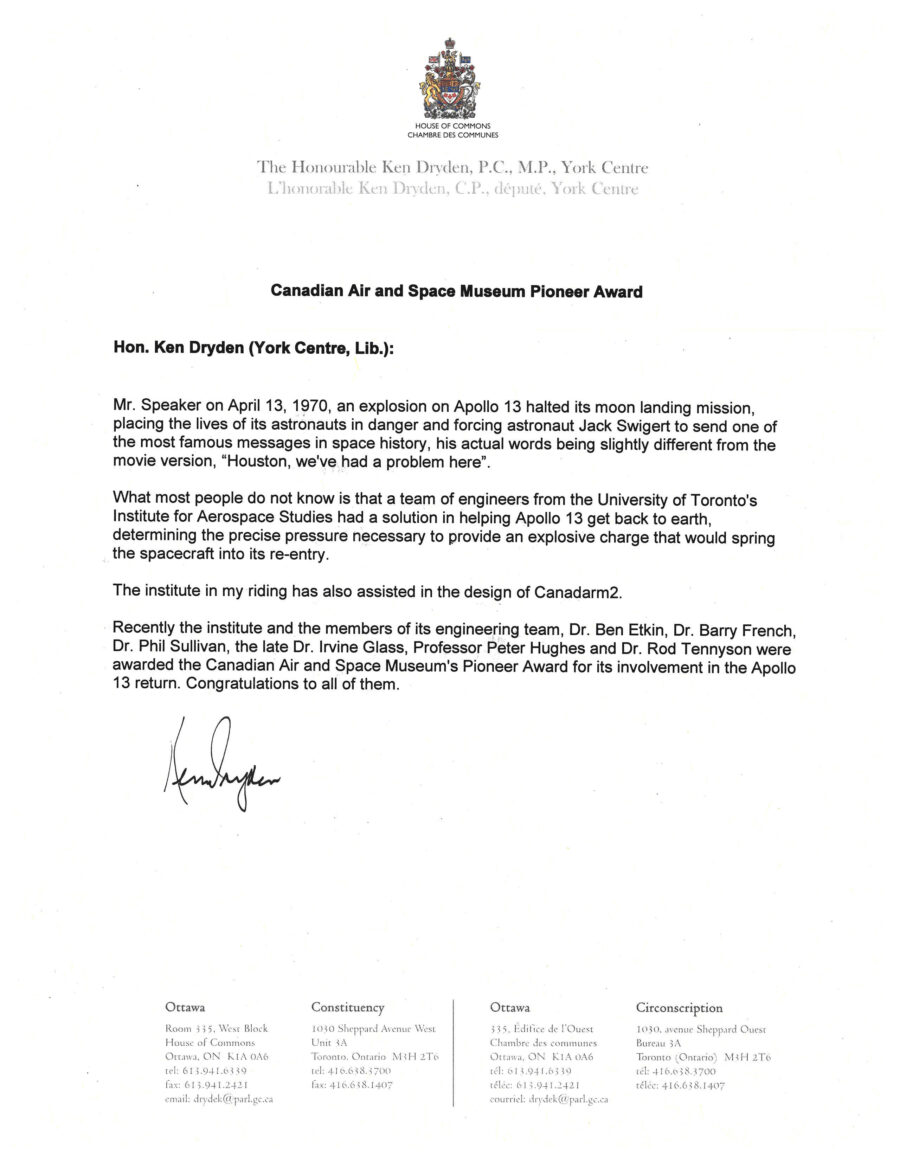
In 2011, Apollo 13 astronaut and Lunar Module pilot Fred Haise visited UTIAS and met with the remaining professors who helped to rescue the three Apollo 13 astronauts.
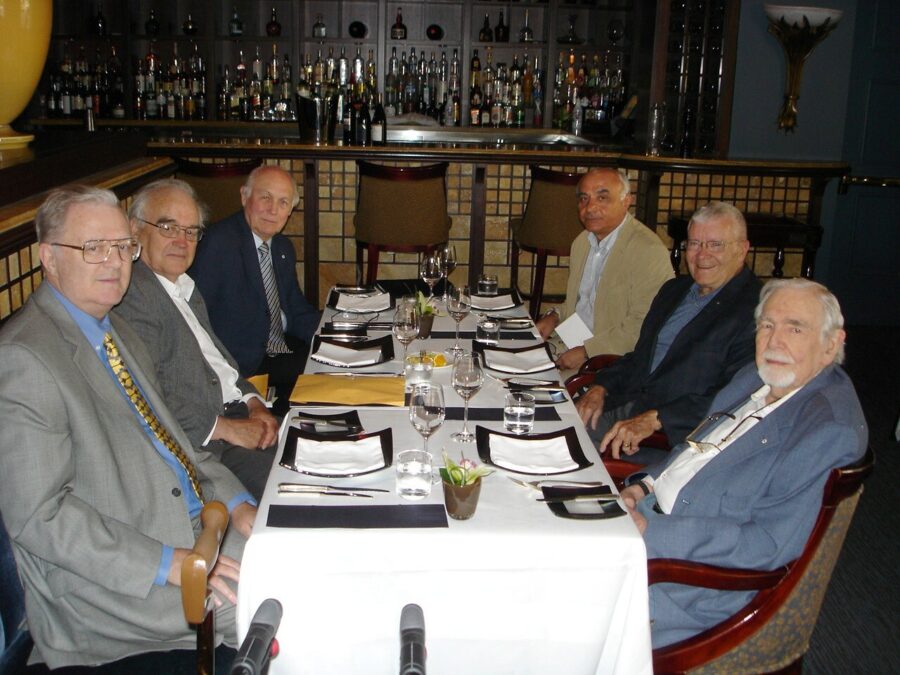
Dinner with Apollo 13 Astronaut Fred Haise and the remaining UTIAS team members: (From left to right) Professors Emeriti Peter Hughes, Phil Sullivan, and Barry French, Professor Ömer Gülder (UTIAS Associate Director and host of the dinner), Fred Haise, and University Professor Emeritus Ben Etkin, absent Professor Emeritus Rod Tennyson.
After his visit, Fred followed up with an email to Joan DaCosta:
“If you see Professors Sullivan, French, and others of that group who helped out during Apollo 13 you can tell them I use their contribution a lot in my talk. It makes a great example of the great support there was for the program. Both obviously from those directly employed on the Program but others who on the spot volunteered their expertise to make Missions successful. I am sure there are many of these types of stories during the program that will never be heard. But likely none as life or death essential as the Professors work on Apollo 13.”